Know Your Brain: Sodium-Potassium Pump
The sodium-potassium pump (also known as the Na+/K+ pump or Na+/K+-ATPase) is a protein pump found in the cell membrane of neurons (and other cells). Its main function is to transport sodium ions out of the cell and potassium ions into the cell. To understand why this is an important physiological mechanism in neurons, we need to first talk a bit about the concentrations of ions inside and outside of neurons, and why maintaining this concentration is important to how neurons work.
Ions, membrane potential, and action potentials
Ions are atoms that have either gained or lost electrons, and thus have a positive or negative charge. There are many different ions found in the human body, but several specifically play an important role in the function of neurons. These include positively-charged sodium ions, positively-charged potassium ions, negatively-charged chloride ions, and a variety of other negatively-charged ions, sometimes referred to collectively as organic anions (anion is a term for a negatively-charged ion).
These ions are distributed unequally on both sides of the cell membranes of neurons. The sodium and chloride ions are more prevalent outside of the cell, while the potassium ions and organic anions are more prevalent inside the cell. This unequal distribution of ions creates a situation where there is also an unequal distribution of electric charge across the cell membrane. In general, the inside of the neuron is more negatively charged than the outside.
The difference in electrical charge between the inside and outside of a neuron is known as membrane potential. Although it varies, a typical resting membrane potential (the potential when the neuron is at rest and not in the midst of firing) for a neuron is approximately -65 mV, which again reflects that the inside of the cell is more negatively charged than the outside.
One of the special features of neurons is their ability to rapidly and efficiently communicate with one another. To do this, they must be able to not only pass signals between neurons, but also carry signals within themselves—from one end of the neuron to the other. The basis for the signaling that occurs within neurons is a momentary change in membrane potential called an action potential. In an action potential, a massive rush of positively-charged sodium ions flows into the cell and causes membrane potential to change rapidly. Specifically, membrane potential gets closer to zero and eventually—very briefly—becomes positive. This influx of positive sodium ions creates an electrical impulse called the action potential, which then travels from one end of the neuron to the other, and often prompts the release of neurotransmitters.
The function of the sodium-potassium pump
OK, so what does this all have to do with the sodium-potassium pump? Well, let's start with the main function of the pump, which is to transport sodium ions out of the cell and potassium ions into the cell. Typically, these ions do not want to move in these particular directions because doing so would violate the laws of diffusion, which dictate that substances tend to move from areas of high concentration to areas of low concentration.
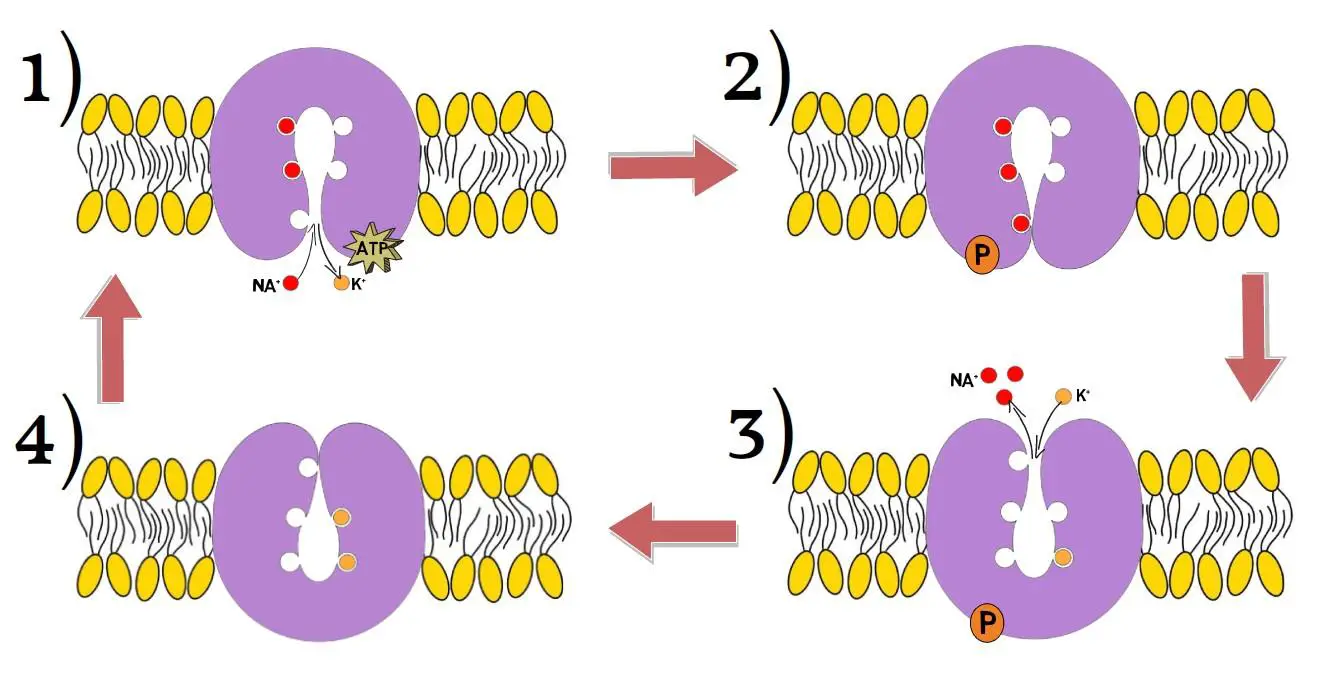
For example, there is already more sodium outside of a cell. Thus, based on laws of diffusion, sodium is not going to be inclined to move towards the outside of the cell since that movement would be from an area of low concentration to an area of high concentration. Therefore, the sodium-potassium pump needs to use energy to transport sodium out of the cell (and potassium into the cell). For this purpose, the pump uses the energy of adenosine triphosphate, or ATP.
All of the details of how the sodium-potassium pump works are not fully clear, but the general process is thought to start with the binding of ATP to the pump. The ATP binding then promotes the binding of 3 sodium ions and the release of two previously bound potassium ions into the cell. ATP is broken down and transfers a chemical group known as a phosphate group to the pump. This process, known as phosphorylation, prompts the pump to undergo a conformational change, or a change in its shape.
This conformational change causes the 3 sodium ions that are bound to the pump to be released into the extracellular fluid, or the area outside of the cell. At the same time, the pump binds 2 potassium ions. The binding of these potassium ions prompts another conformational change, which returns the pump to its previous configuration and starts the cycle anew.
Each cycle of the pump moves 3 sodium ions out of the cell and 2 potassium ions into the cell. Because there is one more positive charge leaving the cell than entering, there is a net loss of positive ions. This makes the resting membrane potential of the cell slightly more negative. This effect, however, is minimal and has little influence on the overall membrane potential of the cell. More importantly, the actions of the sodium-potassium pump help to ensure that sodium ions are more concentrated outside the cell and potassium ions are more concentrated inside the cell. This unequal distribution of ions, combined with other characteristics of the permeability of the cell membrane, creates an environment conducive to an action potential.
Thus, the sodium-potassium pump is critically important to the function of neurons. It helps to maintain ionic concentrations inside and outside the cell that facilitate the ability of neurons to fire action potentials, which is the basis for electrical signaling within neurons.
Learn more: 2-Minute Neuroscience: Sodium-Potassium Pump
References:
Mordecai PB, Yao JPY, Matteson DR. Cellular Physiology and Neurophysiology. 4th ed. St. Louis, MO. Elsevier; 2020.
Purves D, Augustine GJ, Fitzpatrick D, Hall WC, Lamantia AS, Mooney RD, Platt ML, White LE, eds. Neuroscience. 6th ed. New York. Sinauer Associates; 2018.