History of neuroscience: Hodgkin and Huxley
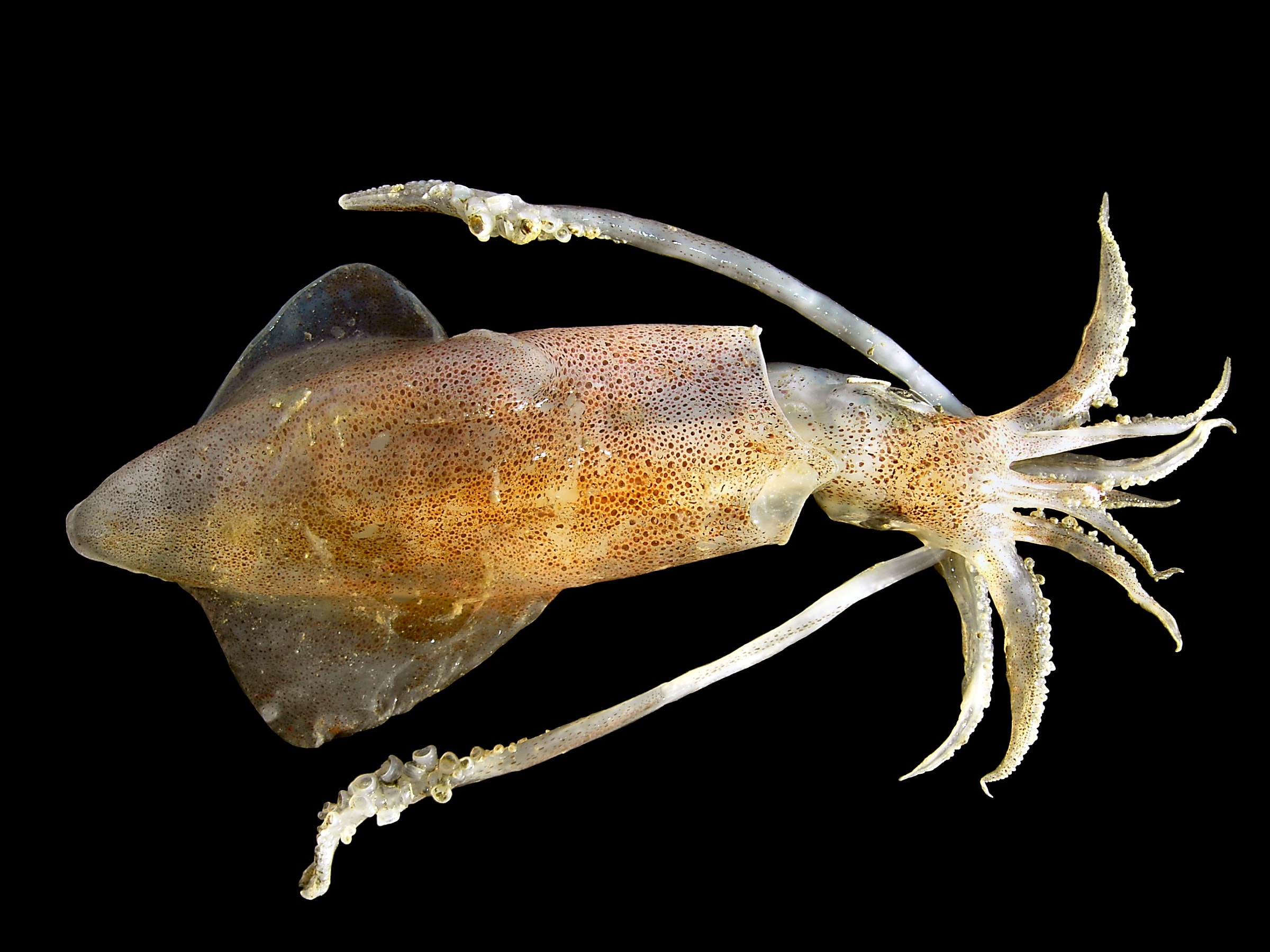
Hodgkin and Huxley used the large axons of the squid to measure voltage changes during an action potential. Image courtesy of hans hillewaert.
By the late 1930s, researchers had come to understand several important things about the conduction of signals within neurons. For example, they knew that signaling within neurons is electrical in nature (as opposed to signaling between neurons, which is usually chemical), and that it occurs in bursts of activity called action potentials. And they knew that action potentials are stimulated by the movement of sodium ions across the neuronal membrane through proteins called ion channels. But the full details of what is going on during an action potential were not made completely clear until Alan Hodgkin and Andrew Huxley started collaborating on the issue in 1939.
What is an action potential?
To understand Hodgkin and Huxley's findings, it helps to have some background on what happens during an action potential. When a neuron is at rest, there are a variety of charged particles called ions that are unevenly distributed inside and outside of the cell. Ions are simply atoms that have either gained or lost an electron; this gain or loss of electrons gives the atoms a negative or positive overall charge, respectively. When a neuron isn't excited, positively-charged sodium ions accumulate outside of the neuron, while positively-charged potassium ions accumulate inside. There are also negatively-charged chloride ions that accumulate outside and organic ions that accumulate inside the cell. A number of mechanisms, including both passive (e.g. diffusion) and active (e.g. the Na-K pump) processes, work to maintain a unequal balance of positively- and negatively-charged particles between the inside and outside of the neuron. This difference in charge is known as the resting membrane potential; typically in neurons it is around -65 to -70 mV, which means that the inside of the neuron is negatively-charged with respect to the outside.
During an action potential, the membrane potential rapidly changes. By Hodgkin and Huxley's time it was already suspected that this rapid change was produced by the movement of positively-charged sodium ions from the outside to the inside of a neuron through ion channels. The influx of positively-charged particles was thought to be the basis for the burst of electrical energy that then proceeded to travel down the neuron as an action potential. However, the extent of the change in membrane potential wasn't known, and the exact role of the different types of ions found in the intracellular and extracellular fluid of neurons had yet to be elucidated.
Voltage clamps and squid axons
One of the difficulties in understanding action potentials before Hodgkin and Huxley's work was that neurons are incredibly small. (At their largest they are about 100 micrometers, but they can be under 10 micrometers. By comparison, a human hair is about 80 micrometers.) Scientists found that the size of the axons in most species made it difficult or impossible to insert a recording device to measure voltage changes during an action potential.
Hodgkin and Huxley got around this problem by studying action potentials in the relatively enormous axons (up to 1 mm in diameter) of the squid. They inserted a fine capillary electrode into the squid giant axon and were able to measure electrical changes within the axon during an action potential. They found that the membrane potential of the neuron actually reversed during an action potential, causing the neuron to momentarily have a positive membrane potential. This rapid reversal of membrane potential was the impetus for the generation of the electrical signal underlying the action potential.
Hodgkin and Huxley also utilized an innovative tool that allowed them to determine the contribution of different ions to the change in membrane potential seen during an action potential. The device, called a voltage clamp, uses electrical stimulation and feedback to set the membrane potential of a neuron at a particular voltage and keep it there. Previous attempts to gauge the exact contribution of different ions to the action potential were stymied by the voltage-dependency of the ion channels involved. Voltage-dependent ion channels open and close when the membrane potential reaches a particular voltage. Thus, because the action potential involves rapid changes in membrane potential, it also involves rapid fluctuations in the opening and closing of ion channels. This happens so quickly that researchers before Hodgkin and Huxley were unable to slow it down enough to get an understanding of what was going on. By using a voltage clamp, Hodgkin and Huxley essentially were able to "freeze" the neuron at particular voltages, which allowed them to gather details on what was happening in the neuron at each stage in the action potential.
Hodgkin and Huxley used the voltage clamp while also manipulating the levels of different ions in the extracellular fluid. In this way they were able to determine the exact contribution of sodium and potassium (and chloride and organic) ions to the action potential. They determined that an influx of sodium ions through voltage-gated sodium ion channels causes a rapid shift in membrane potential, which causes the initiation of the electrical signal that is known as the action potential. Immediately after this change in membrane potential, however, ion channels open that allow potassium ions to flow out of the neuron. This helps the membrane potential to return to its normal level.
Hodgkin and Huxley's work for the first time allowed researchers a step-by-step view of the processes involved in an action potential. Their findings caused interest in electrophysiology to skyrocket, eventually inspiring the development of a more precise form of the voltage clamp known as the patch clamp, which allows for the measurement of current across single ion channels. Perhaps most importantly, however, Hodgkin and Huxley's successful efforts at precisely modeling the action potential would lay the groundwork for a more quantitative approach to biology in the twentieth century.
Schwiening, C. (2012). A brief historical perspective: Hodgkin and Huxley The Journal of Physiology, 590 (11), 2571-2575 DOI: 10.1113/jphysiol.2012.230458