Know Your Brain: Neurons
Neurons are the fundamental cells of the nervous system. They're able to communicate with one another and with cells in other types of tissues (e.g., muscles, glands), and they can carry information from the rest of the body (e.g., sensory information) to the brain. This diverse functionality forms much of the basis for the extensive capabilities of the nervous system.
Estimates vary, but most neuroscientists agree there are about 86 billion neurons in a typical human brain. Each of those neurons forms many connections—about 7,000 on average—with other neurons. This interconnectivity creates large networks of neurons that can transmit messages from one side of the brain to the other in a fraction of a second.
Structure of neurons
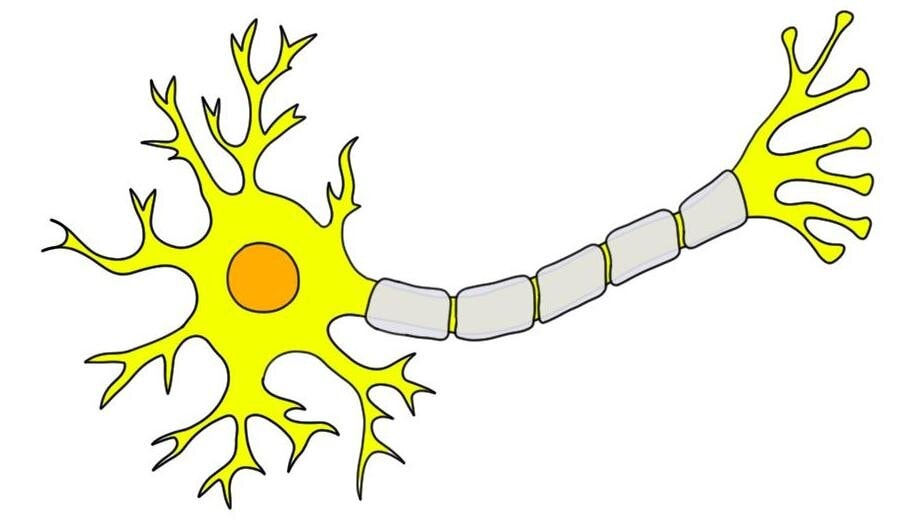
If you look in any neuroscience textbook, you’ll likely see an image of a neuron that looks something like the image to the right. This is the image we’ll be using for our discussion of the neuron in this article. It’s important to mention, though, that while there are neurons in your brain that might vaguely resemble the one in the image, there are many more that don’t.
That’s because neurons come in all shapes and sizes. There are tiny neurons of microscopic lengths and there are neurons that stretch to a meter (about 3 feet) long. There are neurons that have so many processes extending from them that they look like little bushes (Purkinje cells), others that look a bit like baskets (basket cells), and many others. So, while we’ll use an image of the prototypical neuron that is most likely to appear in a textbook in this article, just remember that the brain contains a wide array of neurons, and most of them look quite different from this one.
Introduction to how neurons communicate
It makes it a bit easier to grasp the anatomy of the neuron if we talk about some physiology first. While anatomy involves identifying body parts and where they are found, physiology is concerned with how those body parts work. Thus, to understand the anatomy of a neuron we’ll talk just a bit about how neurons work, and specifically about how they use electrical and chemical signals to pass messages throughout the brain.
The electrical signals, known as action potentials, are essential for neuronal communication. Action potentials are created when charged particles called ions flow into neurons through tiny openings called ion channels. When positively-charged ions enter a neuron, they can create an electrical impulse (the action potential) that can travel down the neuron and cause the release of chemicals called neurotransmitters.
These neurotransmitters leave a neuron (let’s call it neuron A) and then travel to another neuron nearby (neuron B), where they interact with small proteins called receptors. This interaction can lead to the opening of ion channels, and the influx of positively charged ions into neuron B. The influx triggers an action potential in neuron B, and in this way a signal has passed from neuron A to neuron B. The signal can then continue to spread throughout the brain. (Alternatively, the interaction can make it less likely neuron B will fire an action potential, but we won't get into how that happens in this article.)
Those are the (overly-simplified) basics of neuronal communication. When it comes down to it, the most magnificent of our organs is simply an electrical and chemical organic machine.
Anatomy of a neuron
Now that our discussion of neuron function is out of the way, let’s get back to talking about the different parts of a neuron. We’ll start at the far left of the image above and work our way to the right.
Dendrites
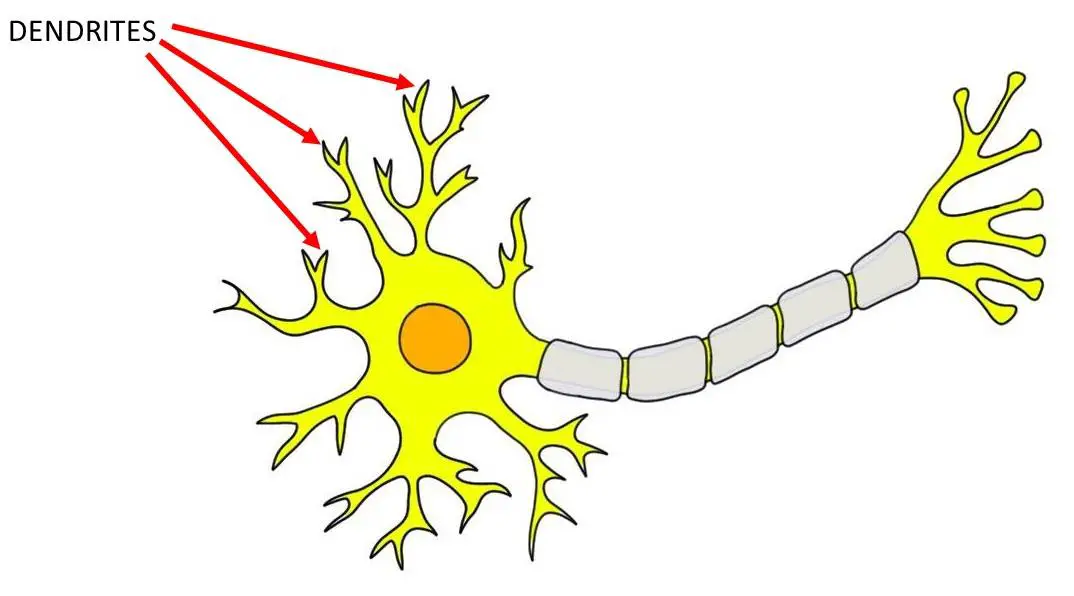
On the left side of the cell in the image to the right you can see a number of tree-like processes jutting out from a circular structure that otherwise resembles the cells you probably learned about in high school. The tree-like extensions are called dendrites.
The word dendrite comes from the Greek word for tree, and the name was used because when early neuroanatomists observed dendrites under the microscope they thought they looked like trees. Dendrites are the part of the neuron that typically receives messages from other neurons. To accomplish this, dendrites are covered with receptors that neurotransmitters are able to interact with. When neurotransmitters attach, or bind, to these receptors, ion channels open, starting the ball rolling on the process described above. Dendrites aren’t the only place on neurons where receptors are present, but they are the most common place to find them.
Cell body
The next part of a neuron that’s important to mention is the part that looks most like a typical cell. This is called the cell body or soma. The cell body contains the nucleus of the cell. You’ve probably learned before that the nucleus is the “control center of the cell.” This description might have been stored away in your brain along with the indelible mantra that mitochondria are the “powerhouses of the cell."
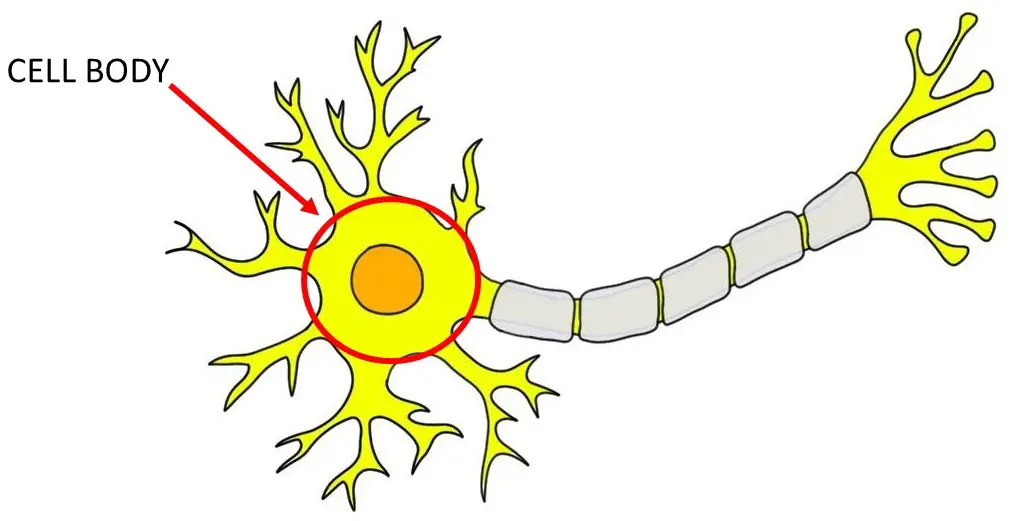
These mnemonics were developed for a reason: they simply summarize the function of these key cellular components. The nucleus contains structures and materials that are necessary for the cell to function properly. Principal among these is the cell’s genetic material: deoxyribonucleic acid, or DNA. In fact, each human cell contains around 2 meters (~6 feet) of DNA, wrapped up into structures called chromosomes. DNA, of course, contains our genes, which act as a blueprint for the production of proteins and the basis for our physical, psychological, and behavioral traits.
The nucleus doesn’t just act as a storage center for DNA. It also controls the expression of genes, a process that determines which genes are “turned on” or “turned off.” This regulates the synthesis of proteins in the cell and acts to coordinate cell function in a number of ways.
The cell body is also a region where the electrical signals generated from the ion channels in the dendrites begin to build up. In a part of the cell body called the axon hillock, these electrical signals must reach a critical threshold in order for the signal to continue down the neuron.
Axon
If the electrical signals do reach that threshold, they are then sent down the long extension of a neuron called the axon. The axon is the part of the neuron that can account for the drastic differences in the length of neurons. Some axons extend only micrometers, or millionths of a meter, while others can span large lengths of the body as they carry information to and from distant targets such as your feet. In fact, according to some estimates, there are enough axons in the brain to wrap around the Earth 4 times!
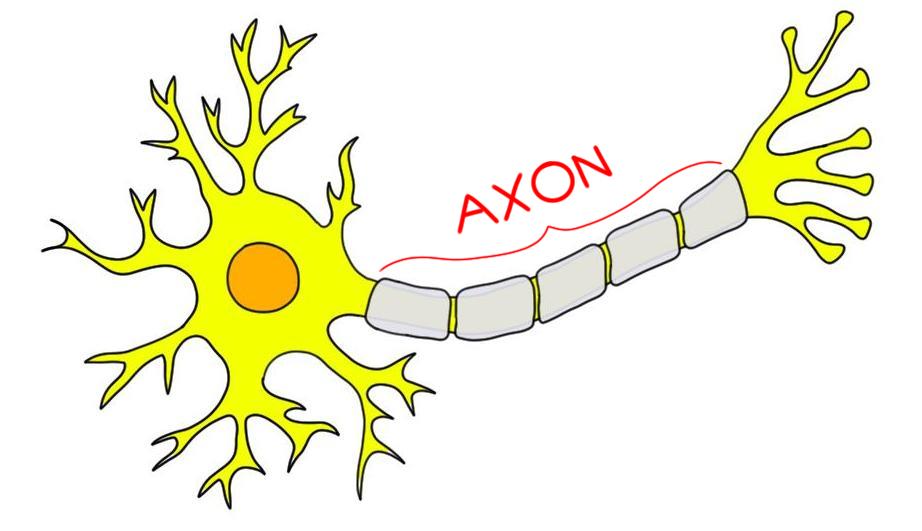
When strong electrical impulses reach the initial part of the axon, they cause the opening of large numbers of ion channels. This prompts a massive influx of positively-charged ions at the beginning of the axon, which triggers an action potential. The axon then serves to propagate the action potential down to the other end of the neuron.
You’ll notice in the image to the right that the axon seems to be covered in another type of material. This material, called myelin, is a fatty substance that surrounds axons and provides insulation. Think of this as serving a similar purpose to the rubber insulation on the electrical cords in your home. The rubber insulation keeps the electricity in the cord, which keeps the electrical signal from escaping before it reaches the device you’re trying to power up. Myelin serves a similar purpose, as it prevents action potentials from decaying as they travel down the axon.
You’ll also see from the image of our neuron that there are intermittent gaps in the myelin. These little gaps are called nodes of Ranvier (named, like so many things in neuroscience, after the neuroscientist who first identified them). The nodes of Ranvier are also critically important for allowing action potentials to pass down a neuron, as even though the insulation is missing here it is replaced with a large number of ion channels. When an action potential reaches a node of Ranvier, ion channels open and allow more positively-charged ions to enter the cell. This acts to rejuvenate the action potential, causing it to speed up and continue moving down the axon.
Because myelin has a white tint, the axons in the nervous system that are wrapped in myelin make up the white matter of the brain. The cell bodies and everything else that’s not myelinated (including some axons, as myelin isn’t found on all of them) make up the gray matter of the brain.
Axon terminals
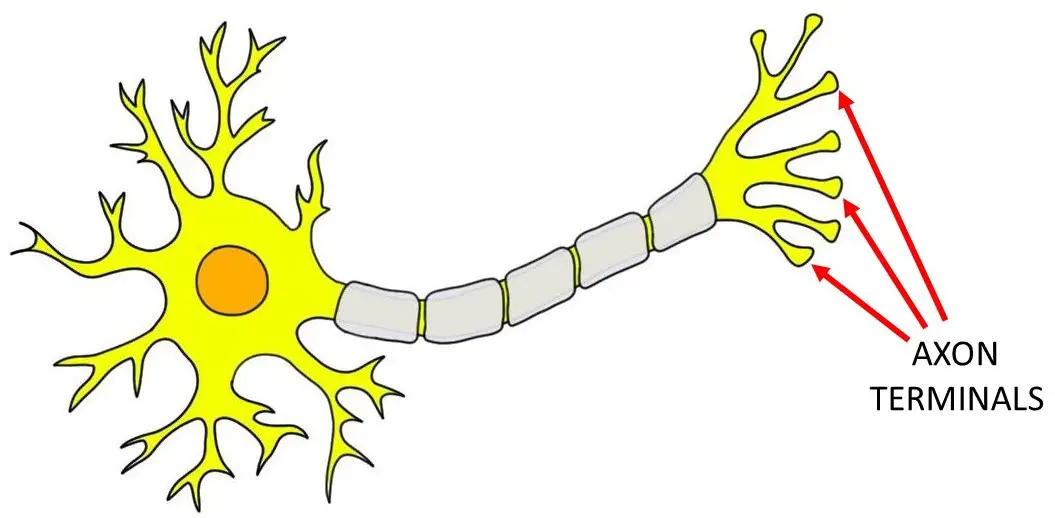
At its end, an axon branches out again into multiple processes. These processes terminate in small swellings called axon terminals or synaptic boutons (boutons is French for buttons, and we’ll learn more about what synaptic means below). The axon terminals are situated close to the dendrites of other neurons. In fact, there is generally only a space of 20-40 nanometers (a nanometer is a billionth of a meter) between the axon terminal of one neuron and a dendrite of another. A human hair is typically around 80,000-100,000 nanometers wide. So, the space between neurons is very, very, very tiny.
This space is called the synaptic cleft. The neuron that precedes the synaptic cleft (in terms of the direction a signal is traveling) is generally called the presynaptic neuron, while the one that follows it is called the postsynaptic neuron. The entire structure (presynaptic neuron, synaptic cleft, and postsynaptic neuron) is called a synapse. The term comes from the Greek for “join together,” since it refers to the places where two neurons come together.
Back to our action potential traveling down the neuron. When it reaches the axon terminals, it can cause the release of neurotransmitters from the presynaptic neuron. These enter the synaptic cleft and interact with receptors on the postsynaptic neuron. Depending on the neurotransmitter, this can either increase or decrease the likelihood that the postsynaptic neuron will fire an action potential of its own. If it does, then the process described above is simply repeated in the next neuron in line.
Reference:
Purves D, Augustine GJ, Fitzpatrick D, Hall WC, Lamantia AS, Mooney RD, Platt ML, White LE, eds. Neuroscience. 6th ed. New York. Sinauer Associates; 2018.